How Transformers Work
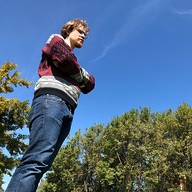
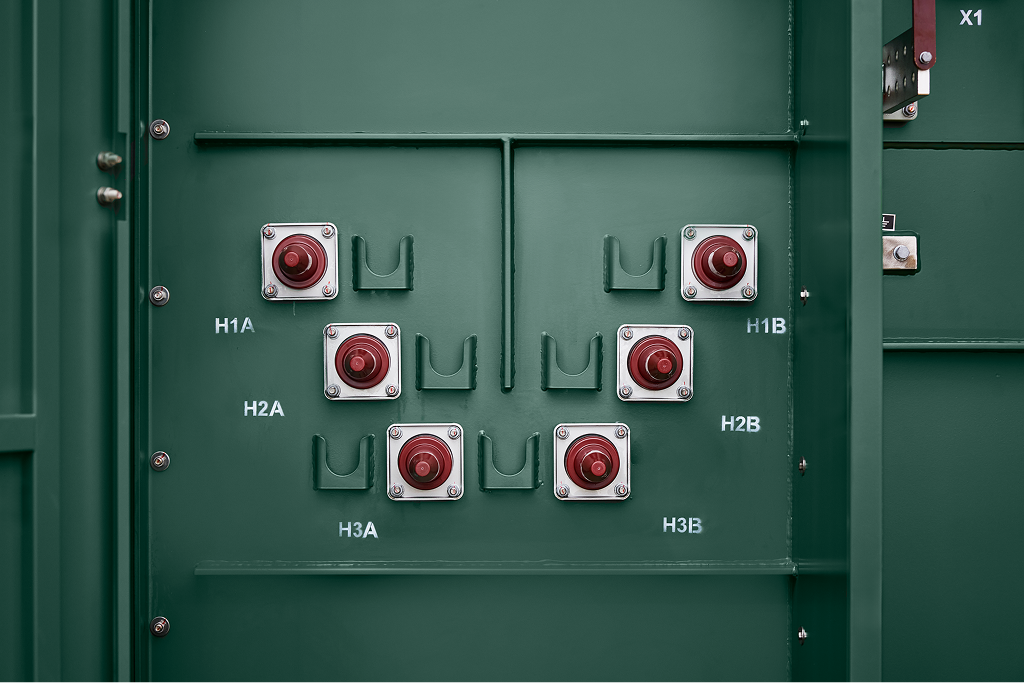
How Transformers Work
This article is the first in a training series where we’ll dive into one of the most important (but often misunderstood) parts of the electrical system: transformers. My name’s JP, I’m a transformer product manager here at Giga Energy, and this series is really designed for our internal sales team, but it’s just as useful for anyone who wants to confidently talk shop with customers.
We’re not trying to turn anyone into an electrical engineer, but we are going to build a rock-solid foundation of understanding. That starts right here.
What Do Transformers Do?
Let’s start with the big picture. At their core, transformers do two things: they either step voltage up or step voltage down. That’s it. And I know that might sound a little too simple, but that basic function enables the entire power grid to work efficiently.
For example, if you’re looking at power generation sites for a wind farm or a coal plant, those generators produce electricity at voltages between 15,000 and 25,000 volts. That’s good, but not good enough for long-distance transmission.
So, to fix this, we run that electricity through a Generator Step-Up (GSU) transformer. That bumps the voltage up to something like 345 or even 500 kilovolts. Why? Because higher voltage means lower current, and lower current means less energy lost to heat as electricity moves down the wire. That’s key for efficiency.
On the other end of the journey, voltage has to be stepped down so that it’s actually usable. Nobody wants 500,000 volts showing up at their house or data center. So, it gets stepped down gradually: first at the substation level for local transmission, and then again for industrial, commercial, or residential distribution. This lets the grid supply power safely to everything from big mining operations to the toaster in your kitchen.
Electrical Basics You Need to Know
Before we delve into the details of how transformers work, let’s get some of the fundamentals out of the way.
Voltage is the difference in electrical potential between two points, so you can think of it like electrical pressure. Current is the rate at which electrons flow, and it’s measured in amperes. Resistance is anything that opposes that flow.
There are also two types of current: direct current (DC), where electrons move in a straight line, and alternating current (AC), where the flow constantly reverses direction. Typical transformers only work with AC, and here’s why: you need a changing magnetic field to induce voltage, and AC gives you that automatically. With DC, the current is steady, so there’s no changing field and, therefore, no induced voltage.
Frequency matters, too. In the U.S., we use 60 Hz, meaning the current changes direction 60 times per second. That’s what keeps the transformer constantly cycling its magnetic field. Then there are phases — most homes run on single-phase power, but industrial sites use three-phase power because it delivers more consistent energy and reduces equipment stress. It’s all about matching the right transformer to the right load.
How Transformers Work
At a high level, transformers are pretty complex. You’ve got two coils of wire (the primary and the secondary) wrapped around a core made of iron or another magnetic material. The magic happens through electromagnetic induction.
Here’s the step-by-step breakdown:
- An AC power source generates alternating current in the primary winding. This current is constantly changing direction and strength.
- That alternating current flows through the primary coil, creating a magnetic field that fluctuates just as the current does. That magnetic field is also called magnetic flux, and this is where Ampere’s Law comes in: it tells us how current generates magnetic fields.
- The iron core focuses and guides that flux. This is all about efficiency — the core ensures more of the magnetic field interacts with the secondary coil.
- The secondary coil, electrically isolated from the first, sits on the same core. Thanks to Faraday’s Law, that changing magnetic field induces a voltage in the secondary coil. The number of turns in the secondary coil determines how much voltage you get out (proportional to the number of turns in the primary coil).
- Finally, you hook a load up to the secondary coil, and boom — you’ve transferred energy from the source to the load without the two ever touching electrically and successfully providing the correct voltage for your load.
That’s the power of magnetic induction. And the best part? No moving parts. This is passive hardware doing all the heavy lifting.
.png)
The Science Behind Transformers
Let's dig a little deeper into key scientific principles, because fully understanding them is crucial when you're talking to a customer — or even just making sense of it yourself.
Faraday’s Law
Faraday’s Law explains how a changing magnetic field can induce a voltage in a nearby coil. It’s the basic principle behind how transformers do their thing — turning magnetism into electricity.
Ampere’s Law
Next up is Ampere’s Law, which explains how the magnetic field itself is created. It states that an electric current flowing through a conductor creates a magnetic field around it. In a transformer, the current flowing through the primary coil generates a magnetic field.
The strength of this magnetic field depends on the amount of current and the number of turns of wire in the coil. This magnetic field is essential because, as we saw in Faraday’s Law, it’s this field that induces the voltage in the secondary coil. Without Ampere’s Law, we wouldn’t have the magnetic fields needed for transformers to function.
Ohm’s Law
Now, let’s talk about Ohm’s Law, which is essential for understanding how voltage, current, and resistance work together in an electrical system. Ohm’s Law states that voltage (E) is equal to the current (I) multiplied by the resistance (R) in the circuit: E = I × R.
This law tells us how much voltage is needed to push a certain amount of current through a particular resistance. In the context of transformers, it helps us understand how voltage and current relate to each other and how changes in one factor (like resistance) can impact the others. For example, when a transformer steps down the voltage, it typically compensates by stepping up the current to maintain power.
The Power Law
So, how much energy are we actually moving? The Power Law has the answer: Power = Voltage × Current. It’s what tells you how much work is getting done — and helps you size your transformer right.
Law of Conservation of Energy
This principle is one of the most important in physics, and you have probably heard about it: energy is never created or destroyed, just transferred. In the case of transformers, it means that the energy entering the primary coil must be equal to the energy leaving the secondary coil, minus any losses due to inefficiencies like heat.
So, if you put in 1,000 volts at 10 amps, you’ll get out 100 volts at 100 amps (assuming 100% efficiency). The power remains roughly the same because the product of voltage and current (Power = Voltage × Current) stays constant, though some energy is inevitably lost as heat in the transformer’s core and wires. The transformer’s job is to convert that power from one form (high voltage, low current) to another (low voltage, high current) while trying to minimize those losses.
Why Step Up Voltage in the First Place?
This is a common customer question, and it’s a great chance to shine. Let’s say you’ve got to move power from a wind farm in West Texas to a factory near Houston. That’s hundreds of miles of transmission line. If you keep the voltage low, your current has to be high to move the same amount of power. But high current means more resistance, and that means more heat. That heat is wasted energy, lost into the air. Plus, more current means you need larger conductors to safely carry the load. Larger conductors cost more and are heavier, which increases the cost and complexity of building and maintaining the transmission system.
By stepping up the voltage, we reduce the current. Lower current = less resistance = less loss. It’s basic physics, but it saves utilities millions in energy loss — and keeps electricity affordable for everyone downstream.
Here’s a real-world example: the Path 56 transmission line runs 850 miles from Oregon to Los Angeles at 500 kV. It’s one of the longest in the U.S. and shows just how far we can push electricity with the right transformer infrastructure. Brazil has a line that runs almost 1,500 miles. None of that would be possible without step-up and step-down transformers.
Wrapping It All Up
If you’ve made it this far, you now have the language you need to talk about transformers with customers.
In future articles, we’ll dig into the different types of transformers (pad-mounted, pole-mounted, isolation, autotransformers, and so on) and when to use each. We’ll also discuss troubleshooting, specs, and how to read nameplates. But for now, this is your foundation.
If you want to keep learning about transformers and don’t want to wait until the next article, then check our resource center to explore technical guides, sizing calculators, and application notes. You can also connect directly with a Giga Energy engineer to get personalized recommendations tailored to your project requirements.
Get a Transformer Quote
Provide us with your specific requirements to receive an immediate quote tailored to your needs.